- Home
-
- Articles
-
-

|
Table of Contents
- 1. Introduction
- 2. Peering at the Lume
- 3. Luminova and the Like
- 3.1 History
- 3.2 How it works
- 3.3 Charging it up
- 3.4 The AfterGlow
- 3.5 Color Codes
- 4. Radio-active Lume
- 4.1 Tritium
- 4.2 Promethium
- 4.3 Radium
- 4.4 Others
- 5. Conclusion
|
Introduction
This article says nothing particularly new, but its purpose is to bring together information as it relates largely to the use and perception of luminous material in watches. A luminous watch is not of much help if we can't see it in the dark, so first we take a brief look at night vision and the performance of the dark-adapted eye. Then we look at the kinds of materials used and examine their properties and application. For those of us that need more, some detailed references are provided throughout the text. We'll use SI units throughout for the light-related stuff:
Common | Scientific |
SI unit | Symbol |
Brightness | Luminous Intensity |
candela | cd |
Brightness | Luminance |
candela/square meter | cd/m² |
Lamp power | Luminous Flux |
lumen | lm |
Illumination | Illuminance |
lux | lx |
Radiation | Activity |
Curie | Ci |
Note: in some industries, the non-SI unit "nit" is used for luminance - symbol "nt". It is numerically equal to 1 cd/m².
|
Peering at the Lume
Our eyes can play tricks on us. On the right, the fatter "hand" appears brighter than the thin one against the same dark backgound (please sit back a little). A little less relevant, but the colors also appear darker as the background lightens. However, the hand brightness and color is exactly the same in each case! The area of the hand and its background influences its apparent brightness and color. Of course, our main interest as watch guys is in the brightness of the lume, rather than its color.
Brightness is measured in cd/m² (candelas per square meter) and its technical name is "luminance". To see our watch hands in the dark, they must be bright enough, right? "Obviously!", I hear you say - but how bright is "bright enough"? A little research soon reveals that there is no exact threshold of brightness below which lume becomes invisible. So, although the eye is capable of detecting an astonishing range of brightness, the actually value of "bright enough" is quite dependent on the background brightness level and how adapted the eye has become to that level. See the following graph which shows sensitivity for both day and night vision.
This graph compares our night (scotopic) with our day (photopic) vision. The vertical axis shows how brightly (measured in lumens) the human eye perceives radiated power (measured in Watts). The retina's cells that are used for photopic vision ("cones") are densely crowded around the center of vision (fovea), giving sharp vision during the day, whereas the cells that are used for scotopic vision ("rods") are not in the direct line of vision but instead more sparsely populated off-center. We can see this at night when the watch lume is faded: it will appear brighter if we look slightly to one side of the watch. Note also that, although the rods not color-sensitive, even the light from watch lume in total darkness is sufficient to fire up a cone cell or two and give some perception of the lume color. You can see more about color perception here.
When the backgound brightness is somewhere between full daytime and pitch darkness, oury eyes adjust to an intermediate type of vision - called "mesopic". It covers the range of luminance from about 0.03 to 3 mcd/m². Note that, for a type of vision, the luminance values represent the average for the whole field of view - not just the watch lume. Actually, the value of "bright enough" that we seek for the lume itself is quite hard to find as related to watches. There are several standards, e.g. DIN 67510, that give a value for a "threshold of visibility" for emergency signs and the concensus for those appears to be 0.32 mcd/m². Finally, a relevant standard was found: "Time-measuring instruments — Photoluminescent deposits — Test methods and requirements" (ISO 17514:2004) and it gives a value of 0.4 mcd/m² for what it calls the "limit of legibility" and these values are for the lume, not including any surrounding areas.
|
Luminova and the like
The majority of luminous watches today use SuperLuminova, Luminova, Lumibrite, etc. These are proprietary compounds which, after "charging" from a source of light, emit an afterglow for several hours. These compounds have largely superceded the use of radioactive paint, due to radiophobic regulations.
History
There is a long history of luminescent pigments. In ancient times, the Chinese found photoluminescent jades and ground them into photoluminescent beads, wine cups or other ornaments. These were collected as precious treasures because of their rarity.
Then, according to an ancient document from the era of Chinese Emperor, Zhao Tai Zhong, the first luminous paint was invented in Japan over 1,000 years ago. The document, (Displayed at the Palace Museum in Taipei, Taiwan) tells of how the emperor heard of a painting which showed a wonderful cow drawn on the wall of a cave. Apparently, the cow could not be seen in the daytime because "it went to a meadow for eating", and "returned to the cave in the evening". Ordered to investigate, one scientist learned that "the cow was painted with special paints made from shells of the sea". Other accounts of the era mention the use of seashells combined with volcanic materials - perhaps pointing to zinc sulfides being formed along with suitable impurities such as copper. The technique of luminous painting was known both to the Chinese and the Japanese and there was some trading of materials between the two countries.
In 18th Century Europe, John Canton prepared a luminous pigment made from oyster shells reacted with sulfur. By the end of the 19th Century, Swiss watchmakers began treating the dials of timepieces with a natural luminescent paint created using the same technique as the early Japanese artists.
The first synthetic luminescent material appeared in Italy in the 17th Century, under the names of 'Stone of Bologna' and 'Sponge of Light'. It was composed of the compound barium sulfide. In more modern times, thanks to the development of science and technology, an artificial photoluminescent pigment was produced. Its chemical composition was a metal sulfide, such as zinc sulfide, doped with copper. The problem was that its luminosity was low and faded quickly. It glowed with a green color for an afterglow time of 1-2 hours, and is still used to this day on toys and some cheap watches. In addition, it was not stable and decomposed quite easily.
Early last decade, a new, brighter photoluminescent pigment with a much longer afterglow time became widely used. its chemical composition is alkaline earth metal aluminate (AEA), made from high purity inorganic materials and doped with "rare earth" metals (lanthanoids). The pigment most commonly used is Strontium Aluminate Oxide doped with "rare earth" impurities such as Europium.
How it works
Light in the range of wavelengths 200-450 nm is absorbed by the pigment and released over a period of time and at a different wavelength. The process is actually called "phosphorescence" which is a special form of photoluminescence. The following two paragraphs transcribed from Wikepedia give more detail (for those WIS's who are into quantum mechanics as well as watches).
Photoluminescence is a process in which a substance absorbs photons (electromagnetic radiation) and then re-radiates photons. Quantum mechanically, this can be described as an excitation to a higher energy state and then a return to a lower energy state accompanied by the emission of a photon. This is one of many forms of luminescence (light emission) and is distinguished by photoexcitation (excitation by photons), hence the prefix "photo-". The period between absorption and emission is typically short, in the order of 10 nanoseconds. Under special circumstances, however, this period can be extended into minutes or hours.
Phosphorescence is the above-mentioned special form of photoluminescence, in which the energy from absorbed photons undergoes intersystem crossing into a state of higher spin multiplicity, usually a triplet state. Once the energy is trapped in the triplet state, transition back to the lower singlet energy states is quantum mechanically forbidden, meaning that it happens much more slowly than other transitions. The result is a slow process of radiative transition back to the singlet state, lasting minutes or hours. This is indeed the basis for "glow in the dark" pigments.
Charging it up
At first, it seems so simple. Hold the watch under a lamp for a few moments . . . and so to bed, for surely the watch lume will glow all night, right? In truth it will, but perhaps not as brightly or as long as advertised. There are a few variables involved in persuading our watch lume to soak up lots of photons:
The dominant wavelength of the illuminating light
The strength (illuminance) of the illumination
The length of time for which the watch is illuminated
Luminous pigments are charged by the UV content of the light, and there is some UV content in most light sources - some more than others. LED UV lamps deliver a good punch, peaking at 365nm or so. Daylight (D65) fluorescent lamps are pretty good, as are the "cool white" or "bright white" variety. Mercury vapor lamps are rich in UV, but sodium lamps are not. The good old-fashioned tungsten lamps have some UV content, but not a lot (sorry, Mr Edison - your time has passed).
A couple of charging rules of thumb:
To an extent, the stronger the light, the quicker the charge and the higher the initial brightness of the lume but the law of diminishing returns does apply.
The longer the time of illumination, the higher the initial brightness of the lume but there is a limit for each level of illumination and that limit is reached more quickly at higher strengths of illumination.
The following table illustrates some of the above points very well. "Constant Load" means charging was continued until the initial brightness of the lume was as bright as it'll get. The light source used was a cool white fluorescent lamp (color temp approx. 4000K) which has a strong UV emission peak at about 400 nm wavelength.
Illumination |
EverGlow Hi® luminance (in mcd/m²) after charging: |
for 15 minutes |
for 60 minutes |
Constant Load |
in f-c |
in lx |
10 min discharge |
60 min discharge |
10 min discharge |
60 min discharge |
10 min discharge |
60 min discharge |
0.9 |
10 |
10 |
3 |
24 |
8 |
36 |
12 |
1.9 |
20 |
26 |
7 |
46 |
12 |
57 |
15 |
4.7 |
50 |
62 |
12 |
82 |
16 |
89 |
18 |
9.3 |
100 |
93 |
16 |
108 |
19 |
113 |
20 |
18.6 |
200 |
120 |
19 |
129 |
20 |
131 |
21 |
46.5 |
500 |
143 |
21 |
147 |
22 |
148 |
22 |
92.9 |
1000 |
153 |
22.5 |
155 |
23 |
155 |
23 |
The above table combines all the data that is usually scattered around in separate places. Several interesting points arise:
Each discharge column of figures shows that, for the light source in question, there is a limit of afterglow brightness with increasing illumination - suggesting that the pigment "saturates" at some illumination level, probably when the photon emission rate equals the photon input rate. According to Arnaud Fily, this is usually called "saturation intensity" which occurs when all the sites that are capable of re-emitting a photon later on have been excited and therefore cannot continue to absorb light. Because of the finite number of sites being charged, there will be also a limit to the amount of re-emitted light (all those sites getting back to their fundamental state).
The charging time has less effect at the higher levels of illumination than at lower levels.
A more subtle effect is seen in the two columns for Constant Load at right. At an illumination of 10 lx, the 60 minute luminance is 1/3 of that at 10 minutes. However, at an illumination of 1000 lx, the 60 minute luminance is only 1/7 of that at 10 minutes - showing that the brighter it is, the quicker it loses luminance - as we will see below.
For what it's worth, for test purposes, ISO 17514 requires a charge of 200 lx from a D65 lamp for 30 minutes or 400 lx for 20 minutes. Do bear in mind that habitual exposure to very high levels of illumination will accelerate the aging of the lume.
|
The Afterglow
The afterglow of today's pigments is both a joy and a disappointment! As we go inside from being out in the sun, the blaze of light from our wrist can be quite stunning. Unfortunately, it does turn quite dull within just a few minutes as can be seen in the following graph from RC Tritec Ltd:
The graph compares the afterglow of Super-LumiNova with Tritium paint. We see that the brightness of Super-LumiNova falls below that of the Tritium paint in a mere 40 minutes. 8 hours and 20 minutes later, its brightness is a just readable 4 mcd/m² (mcd/m² and ncd/mm² are numerically equal). For the Super-LumiNova, the codes are: GL = green, large particle size; C3 = yellow daylight color per ISO 3157:1991. For the Tritium paint, L8.5 indicates a glow brightness of over 80 μcd/g.
Here's another graph - of interest to Panerai Luminor Marina owners:
It's hard to compare this graph with others of its kind because of the peculiar units used for brightness - "ncd/dial" and the use of the word "intensity". Measuring the luminous intensity of the whole dial doesn't tell us much about the brightness of the lume but the values are indeed satisfyingly large. The green line at 30 "ncd/dial" is unexplained but could represent some low limit of legibility. If so, the implication is that the lume on this dial is legible for a stunning 14 hours and 20 minutes!
Finally, here's a Luminova data table from Nemoto & Co, Ltd.
Table Courtesy of Nemoto & Co Ltd. |
LumiNova® Green |
LumiNova® Blue Green |
Conventional Phosphorescent Pigment |
Chemical Identity |
Strontium Aluminate Oxide |
Strontium Aluminate Oxide |
ZnS:Cu |
Body Color |
Light Yellowish Green |
Light Blue |
Yellowish Green |
Avg Particle Size |
1.5-40μm6 |
1.5-20μm6 |
20-40μm |
Excitation by |
200-450nm |
200-450nm |
200-450nm |
Emission at (peak) |
520nm |
490nm |
530nm |
Afterglow Brightness1 |
>300mcd/m² |
>200mcd/m² |
20-30mcd/m² |
Afterglow Extinction2 |
>2,000 min. |
>2,000 min. |
200min. |
Excitation Time3 |
>30 min. |
>30 min. |
4 min. |
Light Fastness4 |
>1,000 hours |
>1,000 hours |
10-24 hours |
Chemical Stability |
Excellent (Except Water) |
Excellent (Except Water) |
Poor to Good |
Specific Gravity5 |
3.6 |
3.9 |
4.1 |
(1) Brightness after 10 min., excitation with a D65 illuminant
for 4 min. at 200 lx.
(2) Time span necessary for afterglow brightness to diminish
to 0.32 mcd/m²(100 times the human eye perception
limit).
(3) Time required for saturation with standard D65
illuminant at 200 lx.
(4) Time required for initial afterglow to drop by 20% after
irradiation with 300W high pressure mercury lamp (accelerated
light fastness test)
(5) In powder form.
(6) Depends on type of LumiNova® (different
particle sizes available).
Ref. Note 4 above, this is saying that if we charge up our lume with lotsa UV for 1000 hrs or more, the lume will be permanently degraded with a 20% loss of brightness.
Ref. Note 6 above, particle size also affects brightness - and here, size matters quite a bit:
Average Particle Size μm | 1.4 |
9 | 19 | 42 |
Glow after 1 hour mcd/m² | 10 |
37 | 50 | 63 |
|
Color Codes
ISO 3157:1991 defines color codes for the daylight appearance of lume, i.e. when it is not glowing:
C1 | White |
C3 | Yellow |
C5 | Greenish Yellow |
C7 | Green |
C9 | Blue-Green |
For example, see the following color samples from RC Tritec Ltd, day time at left, night time at right:
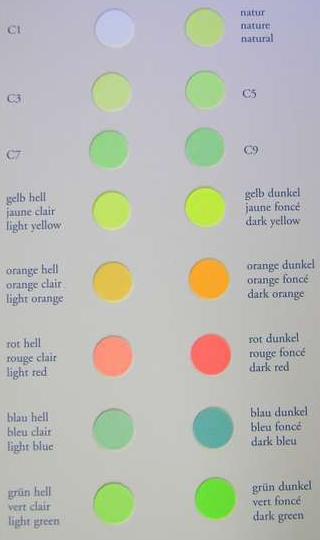 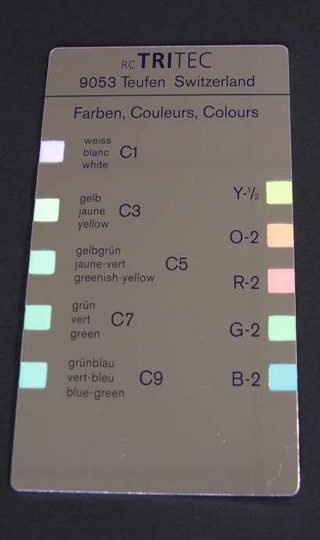
|
Radio-active Lume
The only radio-active material (isotope) used in watches today is Tritium, mostly within tiny glass vials as made by RC Tritec and others. In the past, other materials were used - some with harmful results to watch factory workers. Since the 60's, radio-luminous watches were usually marked in some way to indicate the material and, sometimes, the strength used eg T25 means Tritium emitting a total of 25 milli-Curies (mCi). As we know, there is a considerable variation on how isotopes are symbolized. The Military's H3 and 3H are both strictly incorrect, for example. In the case of Tritium, IUPAC nomenclature allows hydrogen-3 as a descriptor and 3H as a symbol. However, we will use the convention "H-3" in this article because it's easier on your browser and also on my fingers! Much of the following material has been copied from this text-only but excellent article by Paul Frame, of Oak Ridge Associated Universities.The
regulations set forth by the Nuclear Regulatory Commission (NRC) in 10 CFR
30.15 state that an individual does not need a license to possess
timepieces if the following specifications are not exceeded:
(i)
25 millicuries of tritium per timepiece,
(ii)
5 millicuries of tritium per hand,
(iii)
15 millicuries of tritium per dial (bezels when used shall be considered
as part of the dial),
(iv)
100 microcuries of promethium 147 per watch or 200 microcuries of
promethium 147 per any other timepiece,
(v)
20 microcuries of promethium 147 per watch hand or 40 microcuries of
promethium 147 per other timepiece hand,
(vi)
60 microcuries of promethium 147 per watch dial or 120 microcuries of
promethium 147 per other timepiece dial (bezels when used shall be
considered as part of the dial),
(vii)
The levels of radiation from hands and dials containing promethium 147
will not exceed, when measured through 50 milligrams per square centimeter
of absorber: (A) For wrist watches, 0.1 millirad per hour at 10
centimeters from any surface, (B) For pocket watches, 0.1 millirad per
hour at 1 centimeter from any surface, (C) For any other timepiece, 0.2
millirad per hour at 10 centimeters from any surface.
Note
that it is possible to petition the NRC under the provisions of 10 CFR
30.19 to produce timepieces with activities exceeding those in 10 CFR
30.15.
Tritium
Tritium (H-3) is a low energy
beta emitter with a 12.3 year half-life. The low energy beta particles
cannot penetrate the watch case.
For
a short time during the 1970s, tritium containing gas tube light sources (GTLS) were used to
backlight liquid crystal display (LCD) watches. At that time, a typical activity for GTLS
was 200 mCi. The sealed tubes, made of borosilicate glass, were 0.5
to 1.0 cm long, and one or two millimeters in diameter. The inside wall of
the tube was coated with the luminescent material while the tritium was
present as a gas. These days, similar but smaller tubes attached to the hands and dial faces of analog
watches are used with an activity of no more than 25 mCi except by special licensing.
When incorporated into radioluminescent paint,
much lower activities (2 mCi) are employed. The tritium is incorporated into a polymer that also contains the
luminescent material (usually ZnS).
Promethium
Promethium-147
(Pm-147) is a low energy beta emitter with a 2.6 year half-life. It emits
a gamma ray at 121 keV in a small fraction of its decays. The
Pm-147, mixed together with ZnS in a polymer paint, is either an insoluble
oxide or is incorporated into ceramic microspheres. For a watch or clock
containing Pm-147, the activity is approximately 0.05 mCi (50 uCi). The low energy beta particles
cannot penetrate the watch case.
Radium
Radium-226 is an alpha emitter with a 1600 year half-life. It emits a gamma ray at 186 keV. Radium decays into a number of short lived decay products that can usually be expected to be present at, or close to, the same activity as the radium. These decay products (Rn-222, Po-218, Pb-214, Bi-214, Po-214, Pb-210, Bi-210, and Po-210) emit alphas, betas and gamma rays. The invention of radioluminescent paint can be attributed to William J Hammer who mixed radium with zinc sulfide (in 1902), and applied the paint to various items including watches and clock dials. In a case of bad judgment, he failed to patent the idea. Recognizing a good opportunity, a gemologist at Tiffany & Company, by the name of George Kunz, did patent it. Kunz and Charles Baskerville, a chemist, made their paint by mixing radium-barium carbonate with zinc sulfide and linseed oil.
Zinc sulfide was mixed with a radium salt, usually radium sulfate, to yield products containing about 25 to 300 ug [one ug is essentially the same as one uCi] of radium element per gram. The more concentrated preparations (generally containing 215 ug of radium per gram to meet British Admiralty standards) were used on aircraft and ship instrument dials, while lower-grade materials containing about 50 to 100 ug of radium per gram of mixture were used on watches, switch markings, and other devices requiring less critical reading. Impurities were added to the zinc sulfide, approximately: Cadmium, 0.05 per cent; copper, 0.001 per cent; manganese, 0.0002 per cent.
Over time, the intensity of the glow from the paint decreased because of the damage caused to the ZnS crystals by the alpha particles. Since radium-226 has a 1600 year half life, its own decay is not a significant factor. One of the best kept trade secrets of the various manufacturers was how they minimized the inevitable decrease in the luminosity of their paint. In the 1920s, "mesothorium" was sometimes added to the paint - mesothorium is the historical name given to radium-228 (5.8 year half-life). Ra-228 is a beta emitter, but over time it decays into thorium-228 (1.9 year half-life), an alpha emitter. The result is that the intensity of the light from the paint would actually increase over the first five years or so due to the in-growth of the Th-228. The practice of adding mesothorium ended by 1930. Any mesothorium that might have been used will no longer be present because it has long since decayed away.
During the 1920s, the radium paint was applied to clock and watch components in a variety of ways: painting it on with a brush, painting it with a pen or stylus, applying it with a mechanical press, and dusting. The latter procedure involved dusting a freshly painted dial with radioluminescent powder so that the powder would stick to the paint.
The original practice of using the mouth to put a point on a brush is described as follows by Robley Evans: "In painting the numerals on a fine watch, for example, an effort to duplicate the shaded script numeral of a professional penman was made. The 2, 3, 6, and 8 were hardest to make correctly, for the fine lines which contrast with the heavy strokes in these numerals were usually too broad, even with the use of the finest, clipped brushes. To rectify these broad parts the brush was cleaned and then drawn along the line like an eraser to remove the excess paint. For wiping and tipping the brush the workers found that that either a cloth or their fingers were too harsh, but by wiping the brush clean between their lips the proper erasing point could be obtained. This led to the so-called practice of "tipping" or pointing the brush in the lips. In some plants the brush was also tipped before painting a numeral. The paint so wiped off the brush was swallowed."
It might be worth noting that tipping the brushes was not something the dial painters just decided to do. At least in some plants they were actually trained how to do it. In fact, the instructors sometimes swallowed some of the paint to show that it was harmless. It has been estimated that a dial painter would ingest a few hundred to a few thousand microcuries of radium per year. While most of the ingested radium would pass through the body, some fraction of it would be absorbed and accumulate in the skeleton. Later on, Robley Evans would establish a maximum permissible body burden for radium of 0.1 uCi.
As a result of the ingestion of the radium, many of the dial painters developed medical problems of varying degrees of severity. The first deaths occurred in the mid 1920s, and by 1926 the practice of tipping the brushes seems to have ended.
Others
Strontium-90 (yep, the stuff that goes into your bones and stays there forever) has not been used in watches but, in the early 1950s, the U.S. military began to use Sr-90 instead of Ra-226 in its radioluminous personnel and deck markers! Sr-90 is a pure beta emitter (although there can be substantial bremsstrahlung production) with a 30 year half-life. The high energy betas associated with Sr-90 are actually produced by its short-lived decay product, Y-90. Sr-90 and Y-90 are almost always present together in the same activities (i.e., in secular equilibrium).
Strontium had three major advantages over radium: a greater variety of luminescent colors were possible with strontium than radium, the gradual decrease in luminous intensity was minimal because strontium's beta particles did less damage to the zinc sulfide crystals than radium's alpha particles, and the photon (bremsstrahlung) emissions from strontium were less of a safety concern than the gamma rays from radium.
|
Conclusion
This is the final draft of this article. It tries to strike a balance between the trivial and the excessively detailed. If you see any errors or typos, please take the time to email me here  Best Regards,
xpatUSA
| |
|